TUESDAY, 26 MAY 2020
IN RECENT YEARS, developments in genome editing technologies have allowed scientists to manipulate human DNA with increased ease and precision. However, regulatory and ethical frameworks have not kept pace with the technology, leaving scientists without clear guidelines in a field already plagued by debates surrounding the safety and morality of its research. Traditional gene therapy typically relies on a desired gene being delivered to a particular cell type in the patient (often specific cell types, relevant to the disease), and subsequently incorporated into the genomes of those cells. Typically, this is carried out on cells that do not pass on their genetic information to their offspring, termed somatic cells, as opposed to germline cells such as the egg, sperm and zygote. Most controversial are those technologies that introduce heritable genetic changes, termed germline edits.Traditionally, such changes have only been introduced into non-human animals, such as the famous ‘oncomouse’ engineered to be predisposed with an increased likelihood of developing cancer and numerous species of agricultural plant. However, in November 2018, the Chinese scientist He Jiankui claimed to have successfully edited the germlines of two human embryos, altering a gene to introduce HIV resistance. Although the scientific accuracy of this claim is debated, the response from the scientific community has been almost uniformly negative with some even describing the work as ‘monstrous’. It is unsurprising then, that the work has prompted a World Health Organisation (WHO) panel to demand a registry for human genome editing and
some scientists to propose a complete moratorium on this line of research. However, the ease with which modern science can edit genetic material suggests it could be a question of when, not if, we see the rise of germline editing therapies. Has Pandora’s box already been opened?
A short history of genome editing
Arguably, humans have been modifying their environment by genetic engineering since the beginning of civilisation itself. Indeed, evidence for selective breeding of plants and livestock extends back thousands of years before Gregor Mendel introduced the concept of inherited factors, which we now call genes, in the 19th century. Plants or animals with a desired trait- for example the largest fruit or best milk production- would be chosen by farmers to create the next generation in the hopes that their offspring would also exhibit that characteristic or phenotype. Since the genetic makeup of the plants underlays these phenotypes, breeders unknowingly manipulated the genomes of crops and livestock. Indeed, Mendel’s key contribution was to demonstrate the existence of units of inheritance that are transferred from one generation to the next. Adoption of early ideas concerning
heredity and selective breeding contributed to moral catastrophes, such as the eugenics programmes of the early 20th century. However, these ideas eventually grew into the modern field of genetics which forms a cornerstone of modern biological research.
The advent of genetic engineering in the modern sense of the term required a deeper knowledge of genetic material. In 1953, Watson, Crick, Franklin and Wilkins discovered the double helical structure of DNA we know today: a ground breaking milestone which expanded our understanding of how the DNA message can be read and copied. In the genetic theory developed in the 1950s and 60s, genetic information is encoded in DNA. Specific segments of DNA, genes, are copied into RNA which carries these instructions to the cellular machinery that makes proteins, which perform many essential functions within the cell. It is the latter
that give rise to the phenotypes Mendel observed. Thus, the instructions encoded in the human genome influence our shape, size, sex, function and, importantly, dysfunction. This mechanistic understanding of genetics made altering genes to eradicate disease or create new phenotypes seem possible. Quickly writing, editing and designing life was, theoretically, now within the realm of science rather than science fiction.
After the 1960s, genetic engineering progressed rapidly. By 1972, the first man-made [recombinant] DNA had been synthesized by joining together DNA from two viruses. By 1973, the first animal gene had been cloned- a segment of DNA from a frog was
transferred into a bacterium, which then produced the frog protein. In 1976 Herbert Boyer founded the first genetic engineering company, Genentech, which used genetically modified bacteria to create the human hormone insulin. However, for many biologists the true potential of genetic engineering instead lay in editing genetic material in living humans. Gene therapy - editing, removing or replacing faulty human genes - held the promise of eradicating genetic disease.
Many systems for gene delivery were developed in the late 1970s and 80s to deliver DNA into cell nuclei. However, researchers struggled to find methods to control the location of gene insertion. This led to the possibilities of genes not integrating into the targeted cell’s genome at all or integrating but not being ‘read’ by the cell’s machinery to produce a protein, rendering the inserted DNA useless. Even worse, a would-be healthy gene might be inserted in the middle of another important gene, destroying the function of the working gene and creating more problems. In 1972,Theodore Friedman and Richard Roblin co-authored
a paper entitled “Gene Therapy for Human Genetic Disease?” in which they envisaged that genetic diseases in humans could be tackled through the use of gene therapy. The paper itself is seen as a milestone in the field, although its main message is one of caution:
“For the foreseeable future ... we oppose any further attempts at gene therapy in human patients because our understanding of such basic processes as gene regulation ... is inadequate”
This feeling of caution was echoed at the 1975 Asilomar Conference, which established tight guidelines on recombinant DNA technology that were subsequently adopted by the American National Institutes of Health and Food and Drug Administration. Despite these technical and social oppositions, by 1990 the first human gene therapy trial was underway. Due to their ability to enter cells readily, researchers used viruses to deliver the desired DNA. In this case, the treatment temporarily replaced a defective gene in a four-year-old girl suffering from a rare genetic disorder, offering a semi-permanent cure.
However, promising early trials became marked by tragedy. In September 1999, Jesse Gelsinger became the first person to die from a gene therapy treatment after volunteering for a clinical trial. In Jesse’s case, the viral vectors used to deliver the gene into his cells caused a severe immune reaction, resulting in his death four days after injection. In 2002, a clinical trial using gene therapy to combat immune deficiency in children was halted after one subject tragically developed leukaemia. The child was dubbed ‘gene therapy’s first cancer victim’. These deaths plainly signalled that our understanding of Friedman’s “basic processes” was still lacking.
Despite these tragedies, gene editing techniques continued to improve throughout the 1990’s and 2000’s. By the mid 1990s, the next generation of gene therapy technologies were developed, promising safer and more effective future treatments. One of the first of these technologies was zinc-finger nucleases (ZFNs). Unlike previous imprecise techniques, ZFNs could recognise and cut specific short sections of DNA, massively increasing the probability that the transgene gets incorporated correctly at the site of the cut. Eventually viral vectors were also created in which the original viral genome was removed. This meant that the virus would be unlikely to reproduce inside the patient, reducing the chance of an adverse reaction. Furthermore, 2009 saw the discovery of transcription activator-like effector nucleases (TALENs) which could cut the genome with far higher specificity than ZFNs and be created in less time. With increasingly sophisticated tools capable of more precise genome engineering restoring faith in gene editing, by the early nineties, trials of gene therapy started to become more commonplace. By 2010, gene therapy trials were being carried out for diseases ranging from inherited retinal disease to HIV. Despite the use of germline editing in agriculture, the associated risks and ethical debate surrounding germline edits in a live human ensured that they remained firmly within biomedical labs. Despite colossal technical progress since the birth of genome engineering, by 2010 reliable, specific and safe editing of DNA was still a scientific dream. It is perhaps for this reason that the ethical and regulatory issues surrounding germline edits are somewhat absent in the scientific literature. This conversation changed dramatically, however, with the discovery that an ancient bacterial defence system called CRISPR could edit DNA. CRISPR ignited a fire which has since permeated modern molecular biology.
From viral immunity to genome engineering – a bacterial immune system kicks off a new frontier in science
CRISPR has become the shorthand for an elegant gene editing tool that has revolutionised the ability of scientists to alter DNA sequences. Discovered through interdisciplinary collaboration among microbiologists and structural biochemists, the CRISPR system is an ancient defence mechanism used by bacteria to fight off viral intruders. Cas9, the protein component of the CRISPR system, cuts DNA in a programmable fashion guided by a set of RNA instructions provided by the cell or, in the context of genetic engineering, by the researcher. RNA and DNA sequences can be designed and produced in a laboratory relatively cheaply and
quickly, and it is easy to design an RNA sequence that targets a very specific region of DNA. This meant that CRISPR systems could be targeted nearly anywhere, in any genome; a groundbreaking leap forward in technology over previous techniques. Scientists now had a site-specific and accurate method to induce changes in the DNA which promised to be simpler to implement and did not incur the time, expense and difficulty of manufacturing ZFNs or TALENs. Unsurprisingly, It didn’t take long for this system to be adapted for human genomes: by 2013 the lab of Feng Zhang reported that CRISPR was working in mammalian cells, igniting an explosive increase in its usage in answering a huge variety of biological questions. Nowadays, CRISPR is a commonplace technology utilised in many laboratories for purposes ranging from editing genes to help understand the role of specific proteins in the biology of the cell, to engineering disease models in mice to better understand disease. CRISPR has rapidly remodelled the research landscape and changed the way we approach biology for the foreseeable future.
The dark side of genome editing... or is it a bright light?
Every new advancement in genome engineering is accompanied by a debate around safety and ethics. With the advent of CRISPR, it rapidly became apparent that this technology could be used to edit human embryos with much greater ease than previous technologies. While the efficiency of editing with CRISPR is increasing, there remains a possibility for ‘off-target’ effects, a technical term for unintended changes to the genome outside of the targeted region. Some of these changes will do nothing; others may affect genes that influence cancer or other diseases, potentially with devastating consequences. As a consequence, the use of CRISPR in a clinical setting from a technical point of view has been subject to appropriate and highly necessary criticism.
Beyond this, many people question the ethical and moral implications for the concept of our species and its evolution if we begin to edit the inheritable genome as we desire. At the extremes, there remains the anxiety surrounding ‘designer’ babies - offspring whose genomes have been engineered prior to gestation in order for the offspring to exhibit certain desirable traits. The scientific community largely agrees that the prospect of using germline editing to introduce cosmetic changes is still far off. A much more likely usage of germline genome editing is the correction of genetic disorders determined by mutation of a single gene, so called ‘genetic correction’. Disorders such as severe combined immunodeficiency and epidermis bullyosa, a rare and severe skin disorder, are perfect examples of diseases which seem appropriate for clinical approval, as replacing the broken copy of a gene with a functional copy would completely change the quality of life for the affected individual.
When CRISPR-edited human embryos with the potential for transfer into the womb were first reported in 2015, the First International Summit on Human Genome Editing was called. The major conclusion of this meeting, attended by experts from across the world, was that it would be irresponsible to proceed with human genome editing until, at the least, safety and efficacy had been demonstrated, and only used in cases where gene therapy had been deemed sufficiently appropriate and necessary. Many deemed this statement a clear and unambiguous scientific consensus against the usage of these technologies in a clinical setting for the time-being.
Fast-forward three years and a Chinese researcher named He Jiankui nervously approached the podium at the Second International Summit on Human Genome Editing. To a horrified audience, He revealed that his lab had edited the DNA of twin babies born late last year, permanently changing the genome of these children and their offspring to come. In doing so, He breached a myriad of ethical, moral and scientific norms surrounding human germline editing, reinvigorating the debate on human genome engineering, this time on a much more acute sense of urgency.
In the field of genetic engineering, a distinction is often drawn between genetic correction and genetic enhancement, and this distinction often enters ethical discussions about the appropriateness of the use of gene editing technologies. Many scientists would agree that correction is more appropriate ethically. However, He aimed to make the twins resistant to HIV by disabling a gene called CCR5, which is important for HIV to gain entry into cells. Since there was no particular likelihood of the twins contracting HIV, despite their HIV-positive father, in the terms described above, He’s intervention is clearly genetic enhancement.
The trivialness of the genetic changes in the case of the twins demonstrates why extreme caution should be exercised in utilising these technologies. CRISPR is not 100% efficient and consequently one of the twins still retains one functional copy of the CCR5 gene, and will therefore not be immune to HIV. Furthermore, while individuals who lack CCR5 do demonstrate resistance to HIV, some studies suggest its deletion may actually increase the likelihood of particular varieties of cancer as well as infections by tick-borne diseases and West-Nile virus. Furthermore, it has been demonstrated that the naturally occurring CCR5 variant provides protection against the most common strain of HIV but not against the less common X4 strain. Those who attended the International Summit remain unconvinced that whilst He successfully disabled the CCR5 gene there were no off-target effects. Therefore while the changes in the genome of the individuals concerned may protect them from infection to an extent, most experts agree that the risks far outweigh the potential benefits in this scenario.
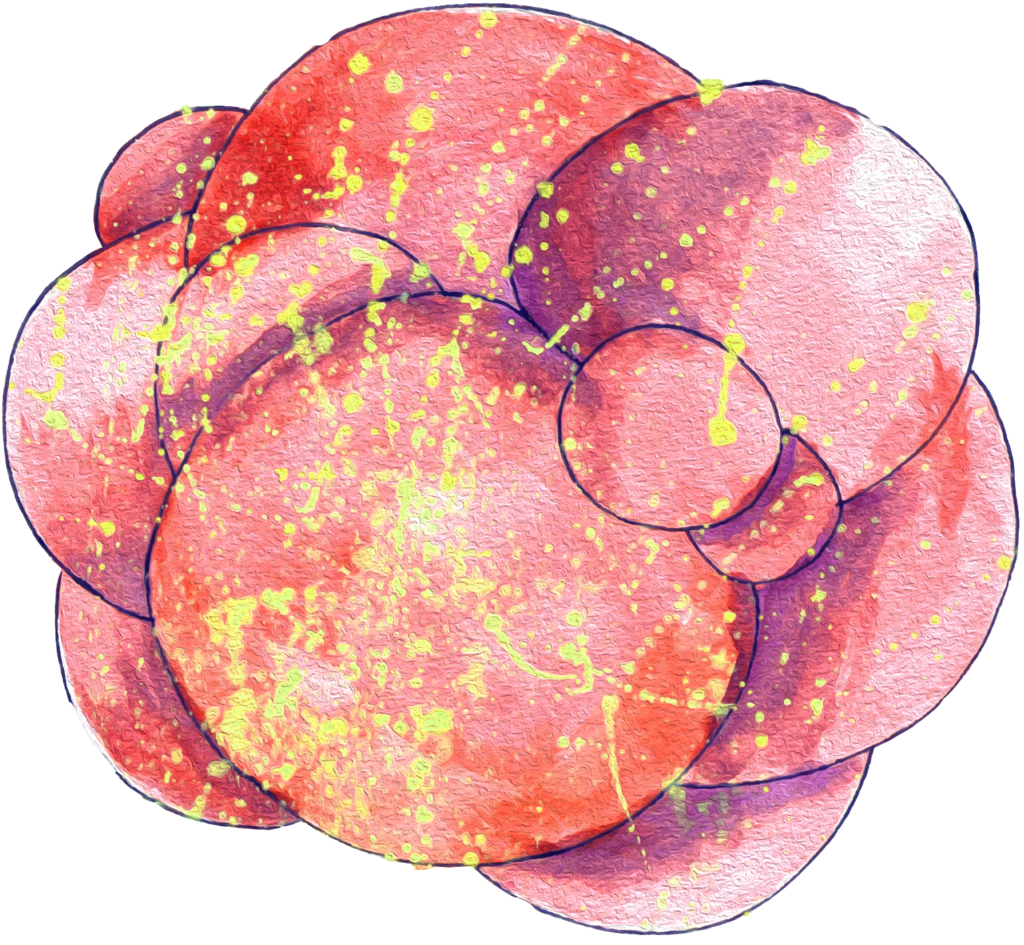
Moving on from CRISPR babies – a future for germline editing?
He’s work crossed numerous ethical and legal lines. Following dismissal from his academic post, He likely faces criminal charges for forging ethical approval and allowing HIV-positive individuals to use reproductive technologies (this is against the law in China). What will happen in the wake of the ongoing national investigation is still unclear. However, there is a broad consensus that the Chinese government bears responsibility for the close monitoring and welfare of the children born from this treatment (allegedly a third is on the way). Indeed China has engaged in public consultation on new draft legislation regarding genome editing.
More striking however is the international discussion provoked by the work presented in Hong Kong. Amongst the most notable was a call by Dr. Eric Lander and colleagues for a five year moratorium on germline editing to allow time for an international regulatory framework to be developed. Lander et al. propose that following the moratorium countries should commit to a three-step review process before any clinical usage of genome engineering. First, a country should distribute a public notice of intent to use human germline editing to allow for discussion of the pros and cons of doing so. Second, countries must engage in rigorous international consultation to satisfy the technical, scientific and medical concerns of the use of the treatment as well as justifying the societal, ethical and moral concerns. Lastly, countries must reach a broader societal consensus within the country in which it will take place on whether the application should proceed at all. This moratorium and model framework have been backed by a number of high-profile bodies, such as the National Institutes of Health, and has been the subject of recent WHO meetings. The latter reinforcing a halt on editing as well as introduction of a registry and canvassing of societal views on editing.
Germline editing is currently illegal in over 30 countries, however in many of these countries approval has been awarded for the use of CRISPR technologies for corrective gene therapy in somatic cells such as those in adult tissues. In 2016, GlaxoSmithKline won ethical approval for Strimvelis, a touted cure for severe combined immunodeficiency due to a mutation in the gene for a protein called adenosine deaminase (ADA). Patients of this devastating and rare disease have severely impaired immune systems and cannot fight off infection. They must remain confined to a sterile environment, resulting in a poor quality of life and low life expectancy. In this treatment, the stem cells which give rise to important immune cells are extracted from the blood. Gene editing is then used to correct the broken copy of the ADA gene. When these cells are returned, the patient can then produce functional immune cells that retain the repaired gene for the rest of their life. While this treatment is only effective for a small number of patients, it represents a safe, ethical, and truly life-changing use of this technology. More recent reports detail that first trials of corrective gene therapy for treatment of two types of sarcoma and multiple myeloma, cancers of connective tissue and the blood respectively, have gained ethical approval in the US.
Routine corrective gene therapy is likely fast approaching, and germline gene editing is, in the opinion of many experts, inevitable. It is clear that Pandora’s box is already open, the question is whether we want to try shut it again. If not, how do we see it becoming a part of our world? What does this mean for our experience as a species?
Andrew Malcolm, Dominic Hall and June Jardin are 1st year PhD students at the Wellcome-MRC Stem Cell Institute. Artwork by Evan Hamilton
IG: @youarelostbecareful